The field of quantum physics has undergone a remarkable transformation in recent years, with groundbreaking discoveries and advancements that have the potential to revolutionize our understanding of the universe. From the intricate dance of subatomic particles to the mysterious realm of quantum entanglement, the realm of quantum physics continues to captivate scientists and the public alike.
Applications of Quantum Mechanics in Modern Technology
Quantum Mechanics in Semiconductor Devices
Quantum mechanics has played a pivotal role in the development of semiconductor devices, which form the backbone of modern electronics and technology. The behavior of electrons in semiconductor materials is governed by the principles of quantum mechanics, and understanding these principles has led to the creation of transistors, integrated circuits, and a wide range of electronic devices that have transformed our daily lives.
- Transistors: The transistor, a fundamental building block of modern electronics, relies on the quantum mechanical properties of semiconductors to control the flow of electric current. The ability to manipulate the behavior of electrons in these materials has enabled the creation of smaller, more efficient, and more powerful electronic devices.
- Integrated Circuits: Integrated circuits, which combine multiple transistors and other electronic components on a single chip, are at the heart of almost every electronic device we use today. The miniaturization of these components, enabled by advancements in quantum mechanics and semiconductor technology, has been a key driver behind the rapid progress of computing power and the proliferation of digital devices.
- Quantum Tunneling: Quantum tunneling, a phenomenon where particles can penetrate through barriers that classical physics would suggest they cannot, plays a crucial role in the operation of many semiconductor devices. This effect is exploited in technologies such as tunnel diodes and resonant tunneling diodes, which have applications in high-speed electronics and microwave devices.
Table 1: Comparison of Transistor Generations
Generation | Year | Transistor Size (nm) |
---|---|---|
1st | 1947 | 3,000 |
2nd | 1959 | 10 |
3rd | 1971 | 10 |
4th | 2007 | 45 |
5th | 2011 | 22 |
6th | 2018 | 7 |
The continuous miniaturization of transistors, driven by our understanding of quantum mechanics, has been a key factor in the exponential growth of computing power and the proliferation of electronic devices in our daily lives.
Quantum Optics and Photonic Devices
Quantum mechanics has also had a profound impact on the field of optics, leading to the development of a wide range of photonic devices that are central to modern telecommunications, information processing, and scientific research.
- Lasers: The operation of lasers, which are essential in fields such as communications, medicine, and material processing, relies on the principles of quantum mechanics, particularly the concept of stimulated emission.
- Quantum Dots: Quantum dots are nanostructures that exhibit unique optical and electronic properties due to their quantum mechanical behavior. They have found applications in displays, solar cells, and quantum computing.
- Quantum Cryptography: Quantum cryptography, also known as quantum key distribution (QKD), uses the principles of quantum mechanics to transmit information in a secure and tamper-proof manner. This technology has the potential to revolutionize the field of cybersecurity.
- Quantum Sensing: Quantum mechanics has enabled the development of highly sensitive sensors that can measure quantities such as magnetic fields, gravitational fields, and time with unprecedented precision. These sensors have applications in fields like navigation, geophysics, and fundamental physics research.
The advancements in quantum optics and photonic devices have been crucial in the development of modern communication systems, information processing, and the exploration of fundamental physical phenomena.
Quantum Materials and Energy Applications
Quantum mechanics has also had a significant impact on the development of novel materials and their applications in energy generation and storage.
- Superconductors: Superconductors are materials that can conduct electricity without resistance, a phenomenon that is explained by the principles of quantum mechanics. Superconductors have applications in power transmission, medical imaging, and particle accelerators.
- Thermoelectric Materials: Quantum mechanics has been instrumental in the design and development of thermoelectric materials, which can convert heat into electricity and vice versa. These materials have potential applications in waste heat recovery, solid-state cooling, and energy generation.
- Quantum Dots in Solar Cells: Quantum dots, due to their unique quantum mechanical properties, have shown great promise in enhancing the efficiency of solar cells. The ability to tune the optical and electronic properties of quantum dots has led to the development of more efficient and cost-effective solar energy technologies.
- Quantum-Based Energy Storage: Researchers are exploring the use of quantum mechanical principles in the development of advanced energy storage technologies, such as quantum-dot-based batteries and quantum-enabled supercapacitors, which could revolutionize the way we store and use energy.
The advancements in quantum materials and their energy applications have the potential to contribute to a more sustainable and efficient energy future.
Key Discoveries in Quantum Entanglement
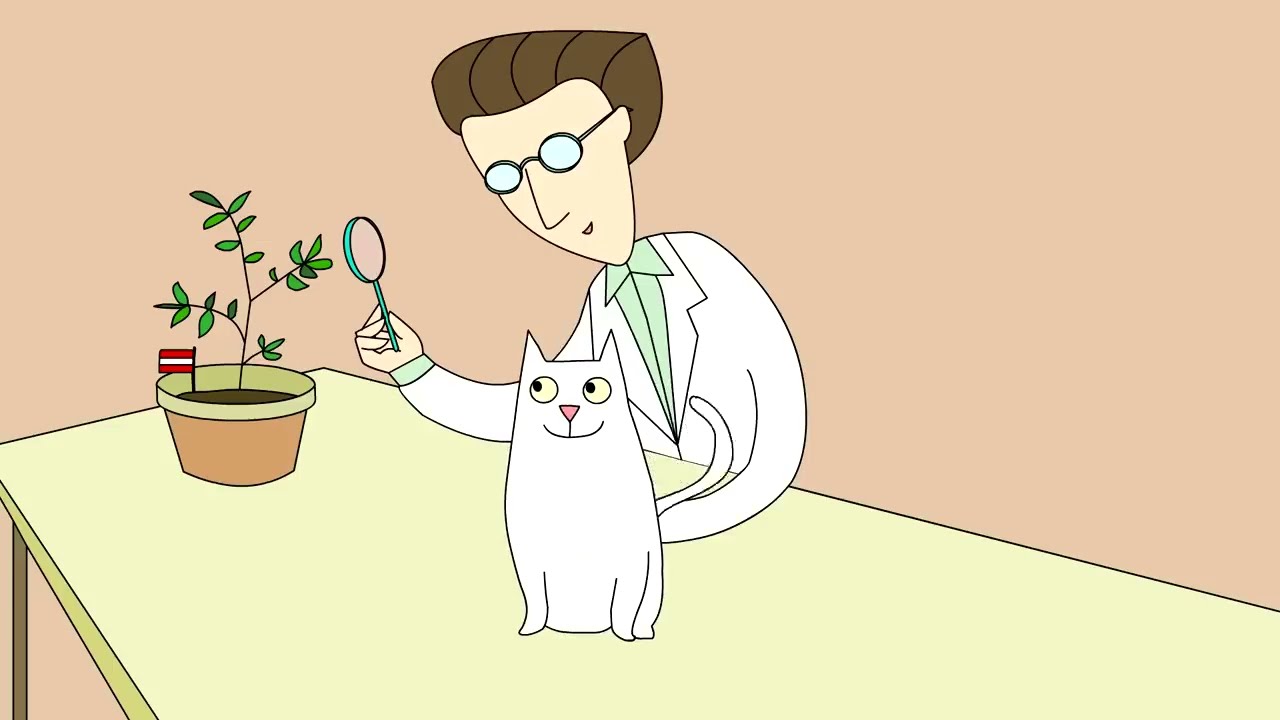
Quantum Entanglement and Its Implications
One of the most intriguing and mystifying aspects of quantum physics is the phenomenon of quantum entanglement. Quantum entanglement occurs when two or more quantum particles become inextricably linked, such that the state of one particle cannot be described independently of the others, even if they are separated by a large distance.
- Spooky Action at a Distance: Einstein famously described quantum entanglement as “spooky action at a distance,” as it seemed to defy the classical understanding of how the physical world should work. This non-local behavior of entangled particles has been a subject of intense study and debate in the field of quantum physics.
- Schrödinger’s Cat: The thought experiment of Schrödinger’s cat, proposed by physicist Erwin Schrödinger, illustrates the counterintuitive nature of quantum superposition and entanglement. The paradox of a cat that is simultaneously alive and dead until observed highlights the challenges in reconciling quantum mechanics with our classical intuitions about the world.
- Experimental Demonstrations: Over the past decades, numerous experiments have been conducted to demonstrate the existence and properties of quantum entanglement. These experiments have provided compelling evidence for the reality of this phenomenon and have led to a deeper understanding of its implications.
Applications of Quantum Entanglement
The remarkable properties of quantum entanglement have opened up a wide range of potential applications in various fields of science and technology.
- Quantum Computing: Quantum entanglement is a crucial ingredient in the development of quantum computers, which have the potential to solve certain computational problems exponentially faster than classical computers. By exploiting the quantum mechanical properties of entangled particles, quantum computers could revolutionize fields such as cryptography, materials science, and drug discovery.
- Quantum Cryptography: Quantum entanglement forms the basis of quantum cryptography, which uses the principles of quantum mechanics to ensure the secure transmission of information. By detecting any attempt to intercept the communication, quantum cryptography provides an unbreakable method of data encryption.
- Quantum Sensing and Metrology: Quantum entanglement can be leveraged to create highly sensitive and precise sensors and measurement devices. These quantum-based sensors have applications in areas such as navigation, gravitational wave detection, and the search for dark matter.
- Quantum Teleportation: The concept of quantum teleportation, where the state of a quantum particle can be transmitted from one location to another, has been demonstrated experimentally. While the teleportation of macroscopic objects remains a distant goal, the principles of quantum teleportation have important implications for secure communication and the development of quantum networks.
The continued exploration and understanding of quantum entanglement hold the promise of transformative breakthroughs in various scientific and technological domains.
Theoretical Advances in Quantum Field Theory
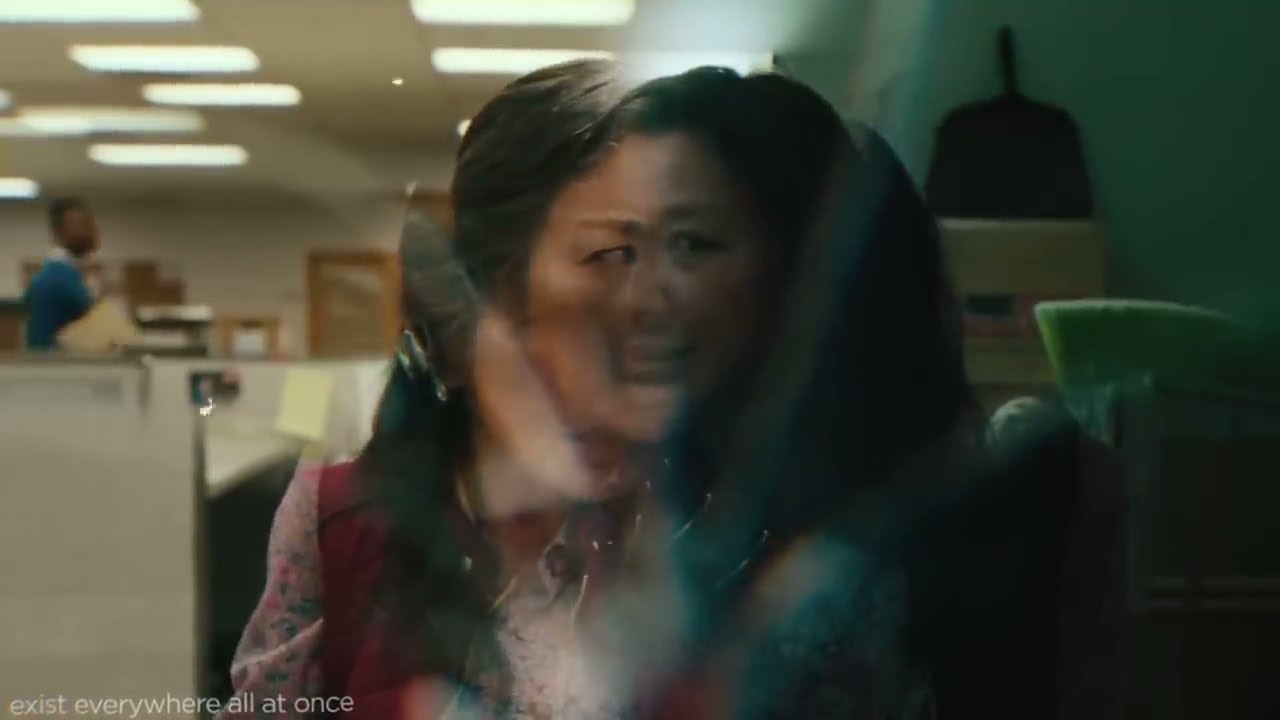
The Standard Model of Particle Physics
The Standard Model of particle physics, developed over the past century, is a comprehensive theory that describes the fundamental particles and the interactions between them. This theory, which is based on the principles of quantum field theory, has been remarkably successful in predicting and explaining a wide range of experimental observations in particle physics.
- Fundamental Particles: The Standard Model identifies the fundamental building blocks of matter, including quarks, leptons, and gauge bosons, and explains how these particles interact through the strong, weak, and electromagnetic forces.
- Gauge Theories: The Standard Model is built upon the mathematical framework of gauge theories, which describe the interactions between particles in terms of the exchange of gauge bosons, such as photons, gluons, and W and Z bosons.
- Quantum Chromodynamics: Quantum chromodynamics (QCD), a crucial component of the Standard Model, describes the strong interactions between quarks and gluons, and has been instrumental in our understanding of the structure of hadrons, such as protons and neutrons.
- Higgs Mechanism: The Higgs mechanism, predicted by the Standard Model and experimentally confirmed with the discovery of the Higgs boson in 2012, explains how particles acquire mass through their interactions with the Higgs field.
Challenges and Limitations of the Standard Model
While the Standard Model has been an incredibly successful theory, it is not without its limitations and challenges:
- Dark Matter and Dark Energy: The Standard Model does not account for the existence of dark matter and dark energy, which are believed to make up the majority of the universe’s energy and matter content. Understanding the nature of these mysterious components of the universe remains a major challenge in modern physics.
- Gravity and Quantum Mechanics: The Standard Model does not include gravity, one of the fundamental forces of nature. Reconciling gravity with the quantum mechanical description of the other forces remains a longstanding problem in theoretical physics.
- Beyond the Standard Model: Physicists continue to search for new physics beyond the Standard Model, which could uncover more fundamental principles and particles that could revolutionize our understanding of the universe.
Advances in Quantum Field Theory
Quantum field theory, the mathematical framework that underpins the Standard Model, has been the subject of ongoing research and development, leading to numerous theoretical advances.
- Renormalization: The development of renormalization techniques, which allow for the systematic elimination of infinities in quantum field theory, has been a crucial breakthrough in the field, enabling precise calculations and predictions.
- Quantum Electrodynamics: Quantum electrodynamics (QED), the quantum theory of electromagnetic interactions, has been extensively studied and refined, leading to some of the most precise predictions and experimental verifications in the history of science.
- Gauge Theories and the Standard Model: The formulation of gauge theories, such as quantum chromodynamics and the electroweak theory, has been a central focus of theoretical advancements, culminating in the remarkably successful Standard Model of particle physics.
- Nonperturbative Methods: Researchers are exploring nonperturbative methods in quantum field theory, which go beyond the traditional perturbative approach and may lead to a deeper understanding of strongly coupled systems and the physics of confinement.
- Quantum Gravity and String Theory: The search for a quantum theory of gravity, which could reconcile the principles of general relativity and quantum mechanics, has led to the development of frameworks such as string theory and loop quantum gravity, which continue to be actively researched.
The ongoing theoretical advancements in quantum field theory and the exploration of new frontiers in particle physics hold the promise of transformative breakthroughs in our understanding of the fundamental nature of the universe.
Quantum Computing: Current Developments and Challenges
The Principles of Quantum Computing
Quantum computing is a rapidly growing field that harnesses the unique properties of quantum mechanics to perform computations in a fundamentally different way than classical computers. The core principles that underlie quantum computing include:
- Quantum Bits (Qubits): In classical computing, information is stored in bits, which can have a value of either 0 or 1. In quantum computing, the basic unit of information is the qubit, which can exist in a superposition of 0 and 1 states, allowing for the representation of more complex information.
- Quantum Superposition: Qubits can exist in a superposition of multiple states, allowing them to represent a vast number of possible combinations simultaneously. This quantum superposition is a key feature that enables the potential power of quantum computers.
- Quantum Entanglement: As discussed earlier, quantum entanglement is a phenomenon where the state of one qubit is inextricably linked to the state of another, even if they are separated by a large distance. Quantum entanglement is a crucial resource for quantum computing.
- Quantum Algorithms: Researchers have developed specialized quantum algorithms, such as Shor’s algorithm for factoring large numbers and Grover’s algorithm for database searching, which can outperform their classical counterparts in certain tasks.
Current Developments in Quantum Computing
The field of quantum computing has seen significant progress in recent years, with both academic and industry players making important advancements:
- Hardware Development: Major tech companies and research institutions have made strides in developing physical quantum computing hardware, including superconducting qubits, trapped ion qubits, and photonic qubits. These efforts aim to create scalable and fault-tolerant quantum computers.
- Software and Algorithms: Alongside hardware development, researchers have been working on the software and algorithms that will enable quantum computers to solve real-world problems. This includes the development of quantum programming languages, compilers, and optimization techniques.
- Quantum Supremacy: In 2019, Google announced that its Sycamore quantum processor had achieved “quantum supremacy,” performing a specific computational task faster than the world’s most powerful classical supercomputer. This milestone demonstrated the potential of quantum computers to outperform classical computers on certain problems.
- Quantum Internet and Communication: The concept of a “quantum internet,” which would use the principles of quantum mechanics to transmit information securely and with high fidelity, is also being actively explored. Advancements in quantum cryptography and quantum teleportation are paving the way for this revolutionary communication network.
Challenges and Roadblocks in Quantum Computing
Despite the significant progress, quantum computing still faces several major challenges and roadblocks that need to be overcome before it can become a practical and widespread technology:
- Qubit Stability and Scalability: Maintaining the fragile quantum state of qubits and scaling up the number of qubits in a quantum computer are major engineering challenges. Researchers are working to improve qubit coherence and develop methods for error correction and fault tolerance.
- Quantum Software and Algorithms: The development of efficient and practical quantum algorithms that can outperform classical algorithms on real-world problems is an ongoing challenge. Researchers are also working on the software infrastructure and programming tools to make quantum computing more accessible.
- Quantum Sensing and Metrology: While quantum computing garners much attention, the applications of quantum mechanics in areas such as sensing, metrology, and imaging are also being actively explored, with the potential for transformative breakthroughs.
- Quantum Computing Ecosystem: The successful development of quantum computing will require the cultivation of a diverse ecosystem, including hardware manufacturers, software developers, domain experts, and end-users. Fostering this collaborative environment is crucial for the widespread adoption of quantum computing.
Despite the significant challenges, the potential of quantum computing to revolutionize fields such as cryptography, materials science, and drug discovery has generated enormous enthusiasm and investment. As the field continues to evolve, the coming years are likely to witness ever-greater advancements and breakthroughs in this exciting frontier of science and technology.
Emerging Trends in Quantum Cryptography
The Need for Quantum-Resistant Cryptography
The advent of large-scale quantum computers poses a significant threat to the security of many of the encryption algorithms and protocols that underpin modern digital communication and data security. This is because quantum computers, with their ability to perform certain computations exponentially faster than classical computers, could potentially break the mathematical foundations of widely used encryption schemes, such as RSA and elliptic curve cryptography.
- Quantum Computing and Cryptanalysis: Shor’s algorithm, a quantum algorithm developed by mathematician Peter Shor, has the potential to efficiently factor large numbers and solve the discrete logarithm problem, which are the foundations of many public-key cryptographic systems. The development of large-scale quantum computers capable of running Shor’s algorithm would render these cryptographic systems obsolete.
- The Quantum Threat: The threat posed by quantum computing to current cryptographic systems has prompted a global effort to develop quantum-resistant or “post-quantum” cryptographic algorithms and protocols that can withstand the attacks of future quantum computers.
Quantum Cryptography and Quantum Key Distribution
One of the most promising approaches to addressing the quantum threat is the field of quantum cryptography, which utilizes the principles of quantum mechanics to ensure the security of communication and data.
- Quantum Key Distribution (QKD): Quantum key distribution is a technique that uses the quantum properties of photons to establish a shared, secret key between two parties. This key can then be used to encrypt and decrypt messages in a way that is resistant to eavesdropping and quantum computing attacks.
- Quantum Entanglement and QKD: Quantum entanglement plays a crucial role in quantum key distribution, as it allows the two parties to generate a shared secret key that is inherently secure due to the fundamental laws of quantum mechanics.
- Practical Implementations: Quantum key distribution systems have been demonstrated in both laboratory and real-world settings, showcasing their ability to provide secure communication channels that are theoretically immune to eavesdropping attacks, even by powerful quantum computers.
Current Trends in Quantum Cryptography Research
The field of quantum cryptography is continuously evolving, with researchers exploring new technologies and protocols to enhance the security and efficiency of cryptographic systems in the quantum era:
- Quantum-Secure Authentication: Researchers are developing authentication schemes that leverage quantum principles to provide secure user verification and access control. Quantum-resistant authentication protocols have the potential to thwart cyberattacks based on quantum algorithms.
- Post-Quantum Cryptography Standards: Standardization bodies and cryptographic experts are working to define and standardize post-quantum cryptographic algorithms that can replace existing vulnerable schemes. These efforts aim to ensure the long-term security of digital communication in a post-quantum world.
- Quantum-Safe Cryptographic Tools: Beyond quantum key distribution, researchers are investigating other cryptographic tools and techniques that can resist quantum attacks, such as lattice-based cryptography, code-based cryptography, and multivariate cryptography. These quantum-safe alternatives offer robust security guarantees against quantum adversaries.
- Interdisciplinary Collaboration: The interdisciplinary nature of quantum cryptography research is fostering collaborations between quantum physicists, cryptographers, mathematicians, and computer scientists. By leveraging expertise from diverse fields, researchers can develop holistic solutions that address the multifaceted challenges of quantum security.
Quantum cryptography holds tremendous promise for ensuring the confidentiality and integrity of sensitive data in the face of rapid technological advancements. By harnessing the laws of quantum mechanics to redefine the foundations of secure communication, quantum cryptography is poised to play a pivotal role in safeguarding our digital future.
Conclusion
In conclusion, the applications of quantum mechanics in modern technology span a wide range of groundbreaking innovations and discoveries. From quantum entanglement and quantum field theory to quantum computing and quantum cryptography, the impact of quantum physics on shaping the future of science and technology cannot be overstated.
Key discoveries in quantum entanglement have revolutionized our understanding of entangled states and their implications for communication and computation. Theoretical advances in quantum field theory continue to drive progress in fundamental physics and particle interactions, offering profound insights into the nature of the universe.
Quantum computing represents a paradigm shift in computational power, with the potential to solve complex problems exponentially faster than classical computers. Despite facing significant challenges, the field of quantum computing is advancing rapidly, fueled by ongoing developments in hardware, software, algorithms, and quantum internet technologies.
Emerging trends in quantum cryptography highlight the critical importance of securing our digital infrastructure against quantum threats. With the rise of quantum-resistant cryptographic schemes and quantum key distribution protocols, researchers are paving the way for a secure communication landscape in the quantum age.
As we embrace the opportunities and navigate the challenges of harnessing quantum mechanics for practical applications, it is clear that the future holds immense potential for innovation and discovery in this fascinating realm of science. By staying at the forefront of quantum research and technology development, we can unlock new frontiers of knowledge and transform the way we interact with the world around us.