The world we perceive, with its familiar laws of cause and effect, its predictable motions and seemingly solid objects, is built on a foundation of classical physics. But beneath this familiar facade lies a realm vastly different, a realm governed by the strange and counterintuitive laws of quantum mechanics. Here, particles behave like waves, probabilities reign supreme, and the very act of observation can alter the course of reality. Quantum mechanics, born from the ashes of the early 20th century scientific revolution, represents a radical departure from classical physics. It arose to explain phenomena that classical physics simply couldn’t account for, like the blackbody radiation spectrum, the photoelectric effect, and the stability of atoms. In the process, it ushered in a new era of understanding, one that forced physicists to confront the limits of their classical intuition and to grapple with a reality that was both bewildering and profoundly beautiful.
Foundations of Quantum Mechanics
Quantum mechanics, or quantum theory, is a branch of physics that deals with the behavior of matter and energy on a subatomic level. It originated in the early 20th century, when scientists were trying to make sense of the strange and seemingly contradictory results of experiments on the atomic and subatomic scale. These experiments revealed that particles could behave like waves, and that the behavior of particles was unpredictable and probabilistic in nature.
The Birth of Quantum Weirdness: From Planck to Heisenberg
The seeds of quantum mechanics were sown by Max Planck in 1900, as he grappled with the enigma of blackbody radiation. Classical physics predicted that a heated object should emit an infinite amount of energy at all frequencies, a prediction that clearly contradicted reality. Planck proposed a radical idea – that energy is not continuous, but instead exists in discrete packets known as “quanta.” This idea laid the foundation for what would become known as quantum theory.
In 1905, Albert Einstein further expanded on Planck’s ideas with his explanation of the photoelectric effect. According to classical physics, increasing the intensity of light should increase the energy of ejected electrons. However, experiments showed that this was not the case – instead, increasing the frequency of light caused the electrons to become more energetic. In order to explain this phenomenon, Einstein proposed that light itself is quantized into particles called photons. This concept would later become a cornerstone of quantum mechanics.
Heisenberg Uncertainty Principle: The Limits of Observation
In 1927, Werner Heisenberg introduced his famous uncertainty principle, which states that it is impossible to know both the position and momentum of a particle with absolute certainty. This concept challenged the fundamental assumptions of classical physics, where the behavior of particles could be predicted precisely if all relevant information was known. Heisenberg’s uncertainty principle demonstrated that the very act of observation had an impact on the behavior of particles, and that there were inherent uncertainties in the nature of reality.
Quantum Theory and Its Applications
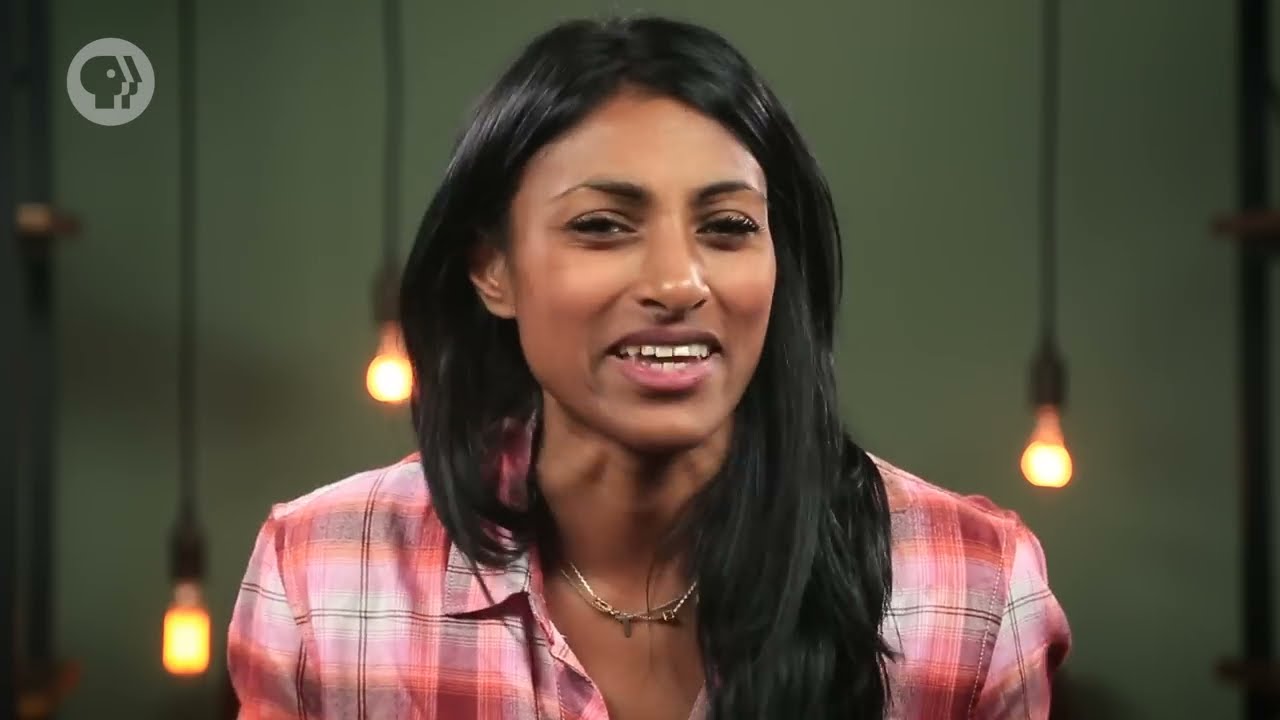
Quantum mechanics has revolutionized our understanding of the universe and has had far-reaching applications in various fields, including technology, medicine, and cryptography. Below are some examples of how quantum theory has been applied in practical ways.
Quantum Teleportation: Beaming Particles Across Space
One of the most fascinating applications of quantum mechanics is teleportation. No, we’re not talking about sci-fi teleportation where people are transferred from one location to another instantaneously. Instead, quantum teleportation involves transferring the quantum state of one particle onto another distant particle. This has already been achieved in laboratories, with particles being teleported over distances of up to 1,400 kilometers.
Quantum teleportation works by exploiting the phenomenon of quantum entanglement (more on that later). Essentially, the quantum state of one particle is transferred onto another entangled particle, creating a perfect replica. This allows for the transmission of information without physically moving particles, which could have significant implications for secure communication and information processing.
Quantum Computing: Harnessing the Power of Superposition
Another groundbreaking application of quantum mechanics is quantum computing. Traditional computers use binary bits (0s and 1s) to store and process data. In contrast, quantum computers use qubits, which can exist in multiple states simultaneously thanks to the principles of superposition and entanglement. This allows quantum computers to solve certain types of problems much faster than classical computers.
Quantum computing has the potential to greatly advance fields such as cryptography, drug discovery, and artificial intelligence. However, due to the delicate nature of qubits and the technical challenges involved in building and maintaining quantum computers, they are still in their early stages of development.
Quantum Computing and Information
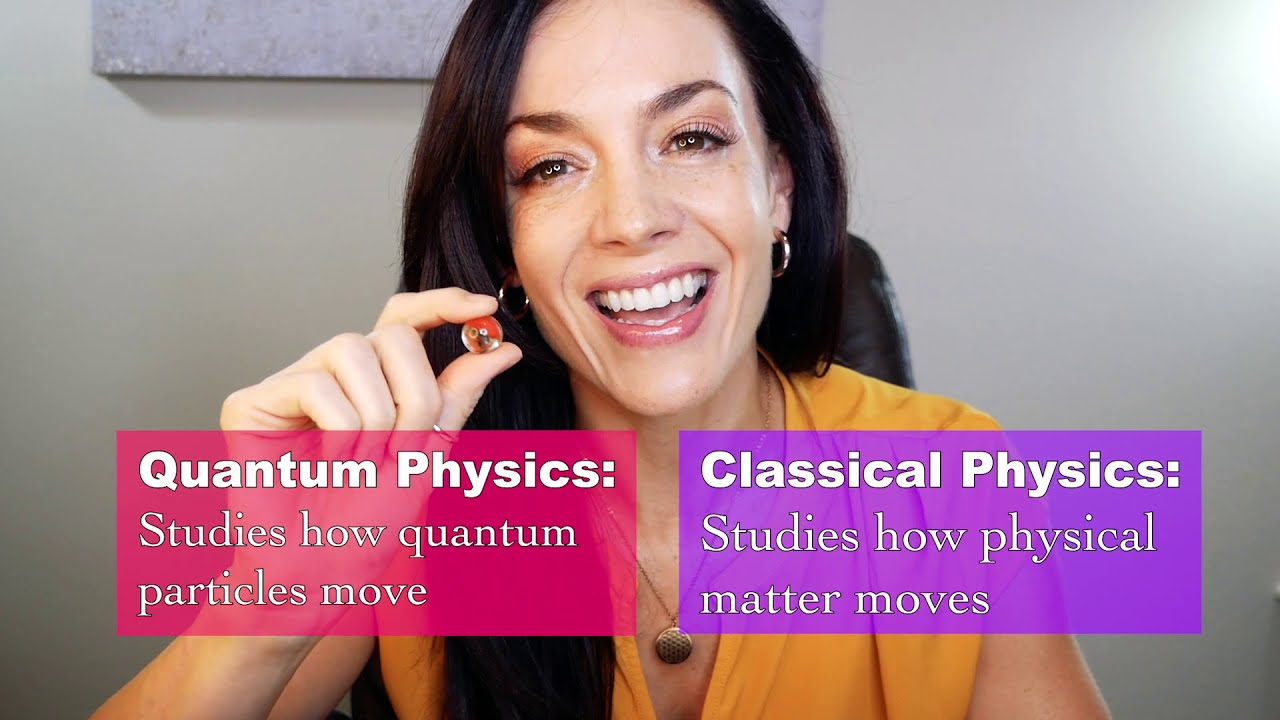
As mentioned earlier, quantum computing is a powerful tool for processing information and solving complex problems. But it’s not just about speed – quantum computing also offers unique advantages in terms of data storage and security.
Quantum Cryptography: Unbreakable Codes
Encryption is an essential part of modern communication and is used to secure sensitive information from prying eyes. However, with the rise of advanced computing techniques, traditional encryption methods may become vulnerable to hacking. This is where quantum cryptography comes in.
Quantum cryptography uses quantum mechanics to generate random codes that are impossible to crack. This is because any attempt to intercept or copy the code would be detected, thanks to the principle of quantum entanglement. This makes quantum cryptography virtually unbreakable, ensuring the security of important data and communications.
Quantum Sensors: Enhanced Precision and Sensitivity
In addition to its computational power, quantum mechanics also offers new possibilities for sensing and measurement. By exploiting the properties of superposition and entanglement, quantum sensors can achieve higher levels of precision and sensitivity compared to classical sensors.
For example, quantum sensors have been used to detect magnetic fields with unprecedented accuracy, allowing for better imaging techniques in medical diagnostics and geological surveys. They have also been used to measure time with incredible precision, which could lead to advancements in areas such as navigation and GPS technology.
Quantum Entanglement and Teleportation
Quantum entanglement is perhaps one of the most intriguing and mysterious aspects of quantum mechanics. It refers to the phenomenon where particles become “entangled” or connected in such a way that the state of one particle affects the state of the other, regardless of the distance between them.
Spooky Action at a Distance: Einstein’s Objection
The concept of quantum entanglement was first proposed by Einstein, Podolsky, and Rosen in 1935, in an attempt to challenge the validity of quantum mechanics. They argued that if entanglement was indeed real, it would violate the principle of locality – the idea that objects cannot be influenced instantaneously over large distances. This led Einstein to famously describe entanglement as “spooky action at a distance.”
EPR Paradox: Debunking Einstein’s Theories
In the 1960s, physicist John Bell devised a mathematical test known as the Bell inequality to determine whether entanglement was real or just a strange prediction of quantum mechanics. In 1982, the French physicist Alain Aspect performed an experiment that confirmed the predictions of quantum mechanics and violated the Bell inequality, proving that entanglement is a real phenomenon.
This result debunked Einstein’s theories and solidified the concept of quantum entanglement. It has since become an essential tool in various applications, including quantum teleportation and cryptography.
Challenges and Future Directions in Quantum Research
Despite the immense progress made in understanding and harnessing the power of quantum mechanics, there are still many challenges and unanswered questions that remain. Some of the key areas of research within quantum mechanics include quantum gravity, the unification of quantum mechanics and general relativity, and the mystery of dark matter.
Quantum Gravity: Bridging Two Theories
Quantum mechanics and general relativity are two of the most successful theories in physics, but they have yet to be reconciled into a single, unified theory. This is known as the problem of quantum gravity. General relativity describes how large objects like planets and stars interact with space and time, while quantum mechanics explains the behavior of particles at a subatomic level. Combining these two theories would provide a complete understanding of the universe and its fundamental building blocks.
However, the challenge lies in the fact that quantum mechanics and general relativity operate on vastly different scales. While quantum mechanics deals with the microscopic world, general relativity deals with the macroscopic world. Finding a way to bridge these two theories is one of the greatest challenges in modern physics.
Dark Matter: An Elusive Mystery
Dark matter is another major enigma in the field of quantum mechanics. Scientists estimate that only 5% of the universe is made up of visible matter, with the remaining 95% consisting of dark matter and dark energy. Dark matter is believed to be an invisible form of matter that does not interact with light or other forms of electromagnetic radiation. Its existence has been inferred through its gravitational effects on visible matter, but its exact nature remains a mystery.
Understanding dark matter and its role in the universe is a significant area of research in both quantum mechanics and astrophysics. Scientists are using advanced technologies such as particle accelerators and telescopes to study this elusive substance and unlock its secrets.
Conclusion
Quantum mechanics has come a long way since its inception in the early 20th century. It has completely transformed our understanding of the universe and has led to groundbreaking discoveries and innovations in various fields. However, there is still much to be explored and understood in the enigmatic world of quantum mechanics. As we continue to push the boundaries of science and technology, who knows what other mysteries and possibilities quantum mechanics may reveal.